The relevance of the oxygen transfer rate for successful cell cultivation
- Lukas
- 1 day ago
- 3 min read
Explaining the critical process factor oxygen transfer rate for bioprocessing
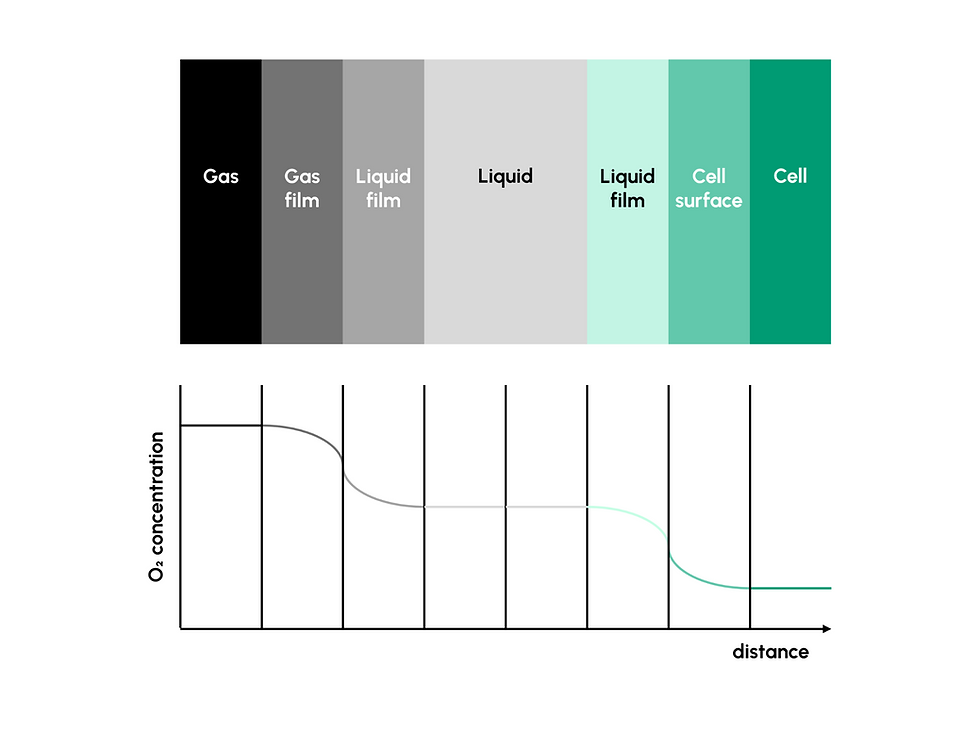
In the development and manufacture of advanced biopharmaceuticals - including cell and gene therapies - cell expansion is a critical step that directly influences yield, quality, and consistency of the final product. Maintaining a viable and productive cell population requires precise control over multiple parameters within the bioreactor environment. Among these, dissolved oxygen (DO) concentration in the liquid phase stands out as a critical process parameter (CPP) that must be tightly regulated to support optimal cell growth, metabolism, and function (1).
Unlike small-molecule pharmaceutical production, where chemical synthesis can occur under relatively static conditions, cell-based production relies on dynamic, living systems that are sensitive to oxygen availability. For aerobic cell cultures, this means oxygen must be continuously delivered and dissolved into the liquid medium to sustain cellular respiration and viability over time. Understanding how oxygen moves from the gas phase into the liquid - and how that movement can be measured, controlled, and optimized - is essential for ensuring process consistency and successful scale-up.
But how can the transport of oxygen into the liquid phase be described? The answer is: with the oxygen transfer rate (OTR) (2).
OTR=kLa*(c*O₂ - cO₂)
The oxygen transfer rate is equal to the oxygen transfer coefficient (kLa) multiplied by the difference between the saturation concentration of oxygen in the liquid phase and the actual oxygen concentration in the medium. The kLa-value consists of two parts. "kL" describes the transport of oxygen into the liquid phase, while "a" represents the surface area of the liquid/gas phase interface.
To modulate the OTR, the phase interface surface a can be increased by reducing the size of gas bubbles in the liquid phase while keeping the gas flow rate constant, increasing the gas flow rate, increasing the stirrer surface, or increasing the oxygen concentration in the gas phase and therefore increasing the saturation concentration in the liquid phase. Together, the kLa-value is a unit of how much mass of oxygen or gas per mass of liquid can be transferred per time (2).
The kLa-value can be used to compare bioreactor systems with each other, independent of the type of gassing (e.g., micro-, macro sparging, or membrane aeration), or mixing (e.g., impeller, liquid jet, or water wheel) (3). This is one of the reasons the kLa-value is also commonly used as a scale-up criterion in upstream process development.
To determine the kLa-value, a common method is the gassing-out method. Nitrogen is introduced to the liquid phase to strip the liquid phase (e.g., water) of oxygen. After the dissolved oxygen concentration does not drop any further, oxygen-containing gas (e.g., air) is introduced into the system, and the increase in dissolved oxygen is monitored with, e.g., dissolved oxygen sensors until the saturation concentration c*O₂ is reached again (4).
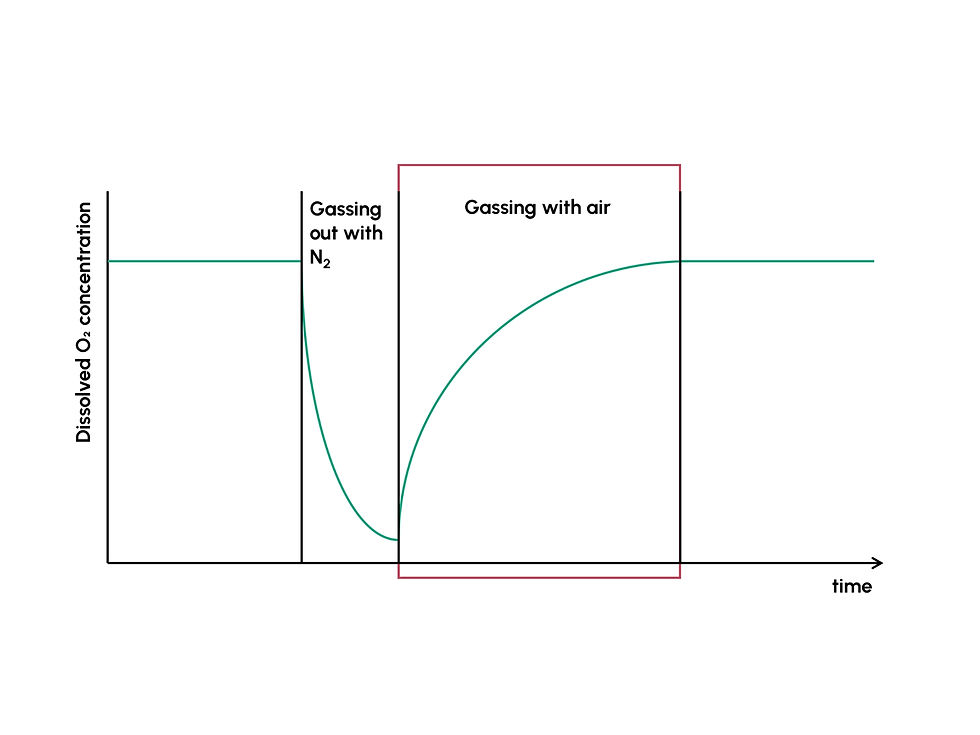
Plotting the natural log of the difference between saturation concentration cO₂ and measured dissolved oxygen concentration cO₂ over time, a linear increase in ln(cO₂ - cO₂) can be observed over time for the period between sparging with air after stripping with N₂ (lowest dissolved oxygen concentration) and reaching the saturation concentration c*O₂ again (red box). The slope of this linear increase is the negative kLa-value. The higher the number (kLa-value), the faster the matter transport. Keep in mind that a change in volume of the liquid phase, stirrer speed/rotational speed, gas flow rate, or oxygen concentration in the gas can massively influence the kLa-value when comparing different bioreactor systems.
Commonly, the kLa value is used to describe and compare stirred tank bioreactors, but it is also applicable to other mixed bioreactor systems like the CellScrew®. For this novel bioreactor system, rotational speed, system size, and oxygen concentration in the gas phase are the most principal factors in manipulating the kLa-value to control. Like other bioreactor systems, the kLa-value can be utilized as a scale-up criterion. For the CellScrew®, the kLa-values were obtained via the method described above, and oxygen transfer was determined to be comparable to a stirred tank reactor in mammalian cell culture, providing optimal cell growth conditions. Find more information about the cultivation conditions in our Application Notes.
References
Xu, WJ., Lin, Y., Mi, CL. et al. Progress in fed-batch culture for recombinant protein production in CHO cells. Appl Microbiol Biotechnol 107, 1063–1075 (2023) https://doi.org/10.1007/s00253-022-12342-x
Chmiel H, editor. Bioprozesstechnik. 3., neu bearb. Aufl. Heidelberg: Spektrum, Akad. Verl; 2011
Karimi A, Golbabaei F, Mehrnia MR, Neghab M, Mohammad K, Nikpey A, et al. Oxygen mass transfer in a stirred tank bioreactor using different impeller configurations for environmental purposes. Iranian Journal of Environmental Health Science & Engineering. 2013;10.
Vorlop J, Lehmann J. Scale-up of bioreactors for fermentation of mammalian cell cultures with special reference to oxygen supply and microcarrier mixing. Chemical Engineering & Technology - CET. 1988;11:171–8.
Comentarios